What is ECC RAM? Differences Between ECC and Non-ECC Memory
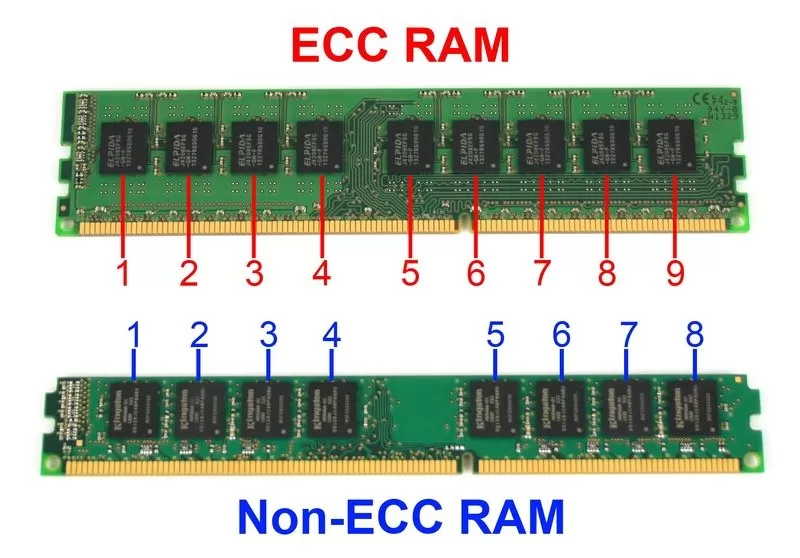
What is ECC RAM? Differences Between ECC and Non-ECC Memory, Memory Ranks, and RAM Selection Guide
Whether you are operating a large data center server or constructing a gaming-oriented PC, selecting the appropriate RAM (memory) is essential for your system's performance and stability. Should you opt for ECC memory, or is standard (non-ECC) memory adequate? What is memory rank? Furthermore, what implications do single rank and dual-rank RAM modules carry? When upgrading your PC or server, these technical terms can often be perplexing.
will address fundamental questions such as what ECC RAM is and the distinctions between ECC and non-ECC RAM. We will elucidate the structure of memory modules under the topic of memory rank, comparing single-rank and dual-rank RAM. Additionally, we will examine the factors influencing your memory selection while addressing how to choose RAM capacity and speed. Finally, we will explore user experiences through Samsung RAM reviews, highlighting leading memory manufacturers, discussing the advantages of HPE RAM and enterprise memory solutions, summarizing information from Micron Crucial RAM reviews, and evaluating the performance of SK Hynix RAM. By the conclusion of this article, which presents technical details in an accessible manner, you will possess a clear understanding of what to consider when selecting the most appropriate memory for your requirements.
What is ECC RAM?
ECC is an acronym for Error-Correcting Code, which refers to Error-Correcting Coded Memory. ECC RAM is a specialized form of memory that enhances system stability by identifying and rectifying data errors that may arise within memory modules. In standard (non-ECC) RAM modules, bit errors in data cannot be directly corrected, potentially leading to erratic system behavior or, in rare instances, crashes. Conversely, ECC RAM incorporates additional error-checking bits for each data word, enabling it to automatically correct single-bit memory errors during operation. This capability is particularly valuable for mitigating the risk of crashes caused by memory errors, especially in critical systems such as servers and workstations.
How does ECC memory accomplish this? In ECC modules, an additional 8 bits of information are stored for each 64-bit block of data, resulting in a total data path of 72 bits. An error-checking code is generated when data is written to memory, and this code is stored in the supplementary 8-bit area. When data is subsequently read from RAM, the system calculates a new check code and compares it to the previously stored code. In the event of a mismatch, the ECC mechanism detects the error and corrects it immediately if it is a single-bit error, or at least ensures that the system recognizes the issue and issues a warning if multiple bits are affected. In this manner, ECC RAM mitigates the adverse effects of minor corruptions that may occur in memory cells over time, such as bit errors caused by cosmic rays, electrical interference, overheating, and other factors.
The primary distinction between ECC memory and non-ECC memory lies in ECC's error detection and correction capabilities. This difference is also evident in the design of the memory module: ECC DIMMs incorporate an additional memory chip (typically one more chip per module than non-ECC modules). We have outlined the key differences between ECC and non-ECC RAM in the table below:
Feature | ECC RAM (Error-Correcting Code Random Access Memory) | Non-ECC RAM (Standard) |
Error Rectification | Automatically identifies and rectifies single-bit errors. Detects, but is unable to correct, double-bit errors. | There is no hardware error correction mechanism; consequently, errors may remain undetected, potentially leading to data corruption. |
Reliability | It provides exceptional reliability, making it ideal for mission-critical servers, financial computing, and scientific research systems. | It is generally acceptable for daily use, though it is more appropriate for non-critical tasks. System stability is not as assured as with ECC. |
Performance | There is a minimal performance penalty (slightly increased latency) associated with the error-checking mechanism, indicating that ECC memory may be marginally slower than its non-ECC counterparts. | It provides the highest raw memory performance due to the absence of supplementary control operations. Slightly improved latency can be attained in gaming and comparable applications. |
Price | ECC modules tend to be more costly due to the inclusion of additional hardware and their appeal to a more niche market. Furthermore, high-capacity ECC modules are typically designed as registered (RDIMM). | It is more cost-efficient than ECC. Its substantial production volume and straightforward design render it a prevalent and economical option for consumers. |
Area of Application | ECC memory supports servers, workstations, data centers, and enterprise applications that demand 24/7 stability, including financial transactions, industrial control systems, and scientific research. | Personal computers, gaming consoles, office workstations, and general applications where fault tolerance is not essential. |
Figure: ECC memory modules contain an additional memory chip (the component highlighted with the magnifying glass in the image above) compared to standard modules. This supplementary chip identifies single-bit errors that may arise in the data stored in memory and rectifies these errors during system operation. The utilization of ECC memory, particularly in critical systems such as servers and workstations, is vital for preventing unexpected crashes and ensuring data integrity.
ECC memory enhances reliability; however, it is not a necessity for every system. For instance, users engaged in gaming or routine office tasks often find non-ECC memory to be adequate and more economical. Additionally, not all motherboards and processors are compatible with ECC memory; to utilize ECC, both the motherboard and CPU must support it. In the Intel lineup, Xeon series processors and server motherboards typically offer ECC support, while certain Ryzen processors, particularly Threadripper and EPYC, from AMD also provide ECC compatibility (some AMD motherboards support UDIMM ECC as well). If ECC memory is installed in a system lacking ECC support, the module will generally function, but the ECC feature will be disabled, resulting in performance akin to standard RAM.
In summary, the response to the inquiry regarding ECC RAM is as follows: it is a memory type designed for reliability, featuring error correction capabilities. If your system requires stable operation around the clock and must be resilient to data errors, ECC RAM is the appropriate choice. Conversely, standard RAM may offer marginally enhanced performance and reduced costs.
Differences Between ECC and Non-ECC RAM
We have outlined the concept of ECC above; now let us explore the distinctions between ECC and non-ECC memory in greater detail. The primary difference can be summarized as follows: ECC memory is capable of detecting and correcting errors, whereas non-ECC memory lacks this functionality. This capability provides ECC with a significant advantage regarding long-term system uptime and data integrity. For instance, employing ECC memory in a server farm mitigates even cosmic ray-induced bit flip errors that may arise in machines operating continuously for years, thereby ensuring the reliable performance of critical applications. In contrast, while the likelihood of such an error occurring in a system with non-ECC memory is low, the ramifications can be severe when it does happen, such as a calculation error in a financial transaction or corruption within a database.
Conversely, ECC memory inherently incurs some performance overhead. The ECC code is computed and verified during each memory operation, resulting in an additional memory latency on the order of nanoseconds. In practical applications, the performance disparity between ECC and non-ECC is typically minimal (~2-3% difference); however, in applications where speed is paramount, non-ECC modules may possess a slight theoretical advantage. This explains why high-frequency memory kits generally exclude ECC, as their intended users are gamers and overclockers. ECC memory is designed to operate at more conservative speeds and timings, prioritizing stability.
Ultimately, cost and compatibility are critical factors in the debate between ECC and non-ECC memory. ECC modules are manufactured in limited quantities and command higher prices due to the inclusion of additional hardware. Furthermore, an important consideration when acquiring ECC memory is whether it is registered (RDIMM) or unbuffered (UDIMM). Many ECC modules, particularly those intended for server use, are registered ECC, which incorporates a register buffer on the memory module. Registered ECC memory alleviates the burden on the motherboard's memory controller and facilitates the utilization of a greater number of modules (high capacities). However, these modules are exclusively compatible with server motherboards. ECC memory utilized in desktop environments is typically referred to as unbuffered ECC (UDIMM ECC) and is compatible with specific workstation motherboards. Non-ECC memory in the consumer market is almost invariably unbuffered, allowing users to forgo additional compatibility checks in this context.
In summary, the distinctions between ECC and non-ECC RAM are as follows: ECC memory offers enhanced reliability and error tolerance, albeit at a marginally higher cost and reduced speed; conversely, non-ECC memory is faster and more economical, yet lacks error correction capabilities. The choice between these two options should be guided by your system requirements. For a gaming PC, non-ECC DDR4/DDR5 memory is adequate. However, for a database server, ECC memory is nearly essential.
What is Memory Rank?
The technical specifications of memory modules often include terms such as "single rank," "dual rank," or even "quad rank." What exactly is memory rank? In essence, each 64-bit wide data block created by groups of chips on a memory module is referred to as a rank. A memory module may contain one or more ranks, contingent upon its capacity and design. Each rank functions as an independent memory bank, allowing the memory controller to access 64-bit data. In ECC modules, as previously mentioned, each rank is 72 bits wide, comprising 64 bits of data plus 8 bits for ECC.
To illustrate with a straightforward example, consider an 8GB DDR4 memory module comprising 8 chips. This module is likely single rank, indicating that the memory controller recognizes it as a single 64-bit memory bank. Now, if we examine a 16GB module, assuming the same technology chips are utilized, it would likely be configured as dual rank to achieve the 16GB capacity, comprising two distinct 64-bit memory banks of 8 chips each, totaling 16 chips (with an additional 2 chips if ECC is present). The memory controller can only interact with one rank of the module at a time, although it can alternate between ranks. In practice, a dual rank module functions as if two single rank modules were installed on a motherboard.
Figure: The concept of rank in memory modules. The image above illustrates a single rank module with ECC support, featuring a total data path of 72 bits (channel) comprising 64 bits for data and 8 bits for ECC. Below, a dual rank module is depicted; each rank possesses its own 72-bit data channel, allowing the memory controller to switch one rank to standby (refresh) mode as needed while processing the other rank. As the number of ranks increases, the module's capacity expands (for instance, high-capacity modules with four ranks are classified as Quad Rank), yet this also elevates the load on the memory controller and complicates the design.
The significance of the memory rank concept for users primarily manifests in performance and compatibility. While single or dual rank modules can influence performance, the rank also dictates the total number of modules that a motherboard can support in specific configurations. For instance, certain motherboards may struggle to operate four dual-rank modules effectively, whereas they may function more reliably with four single-rank modules. This is because as the rank number increases, the load on the memory controller for each memory channel escalates, complicating signal integrity.
Is there a performance difference between single-rank and dual-rank modules? This comparison will be addressed in the following section.
Comparison of Single Rank and Dual Rank RAM
The prevailing consensus indicates that dual-rank memory modules deliver marginally superior performance under specific conditions when compared to single-rank modules with equivalent specifications. This enhancement is primarily attributed to a technique known as Rank Interleaving. While the memory controller executes an operation (reading or writing data) on one rank of a dual-rank module, it can simultaneously prepare the other rank in advance. This process minimizes the waiting time between successive memory accesses and reduces the memory's response time, particularly in applications that engage the processor with memory-intensive tasks. Consequently, dual-rank memories can offer increased bandwidth and improved latency under load. Benchmark tests have demonstrated a performance improvement of approximately 3-5% on the dual rank side when contrasting dual rank and single rank memories with identical frequencies and timings. This disparity may be particularly pronounced in systems equipped with multi-core processors and memory-intensive applications, such as those utilizing integrated graphics, data compression tasks, or virtual machines.
Single-rank memory offers distinct advantages. These modules generate less heat due to a reduced number of chips and typically exhibit greater stability at elevated frequencies. Overclockers recognize that single-rank memory operates more predictably when pushing performance limits and can achieve lower latencies. Furthermore, because single rank modules exert less pressure on the memory controller, even in configurations with four modules, the controller can maintain specified speeds without undue strain (for instance, 4x8 GB single rank kits at DDR4-3200 speeds impose less load on the controller compared to 4x16GB dual rank kits under identical conditions). Consequently, opting for single rank memory may be advantageous in certain systems to attain higher memory frequencies or tighter timings.
The primary disadvantage of dual-rank memory modules is the increased load they impose on the memory controller. Dual rank configurations necessitate additional row and column addressing, as well as signal driver load on the controller. Consequently, dual rank variants of the same memory model typically feature slightly looser timings, such as a higher CAS latency (CL) value, compared to their single rank counterparts. Manufacturers adjust the timings in the SPD (Serial Presence Detect) profiles to mitigate the challenges posed by dual-rank modules for the controller. While this adjustment may diminish the performance benefits of dual rank memory to some degree, it does not entirely negate them; indeed, dual rank modules can still offer significant advantages.
Let us examine two memory kits, each comprising 16GB with DDR4-3200MHz 16-18-18 timings. The first kit includes 2x8 GB single rank modules, while the second features 2x8 GB dual rank modules (totaling 16 chips). Theoretically, the dual rank kit can deliver approximately a 5% enhancement in memory bandwidth under optimal conditions. However, this disparity may not be perceptible across all applications; it is likely to be negligible in gaming scenarios, although the increase in frames per second may be somewhat more evident in systems utilizing integrated graphics. Conversely, if you plan to install four modules, opting for a single rank kit may yield a more stable experience by alleviating stress on the memory controller compared to using four dual rank modules.
In summary, the comparison between single rank and dual rank RAM can be assessed as follows:
- Characteristics of Single-Rank Memories:
- Due to the reduced number of memory chips, it generates less heat and is potentially more stable.
- Overclocking and precise timing (low latency) frequently produce superior outcomes.
- The electrical load on the memory controller is reduced, enabling operation at elevated frequencies in multi-module configurations (such as systems with four DIMMs installed).
- The drawback is that it may exhibit slightly reduced performance under heavy memory usage, as it does not provide the same level of bank parallelism as dual rank for equivalent capacity.
- Characteristics of Dual-Rank Memory:
- Thanks to rank interleaving, it can deliver memory performance improvements of up to 3-5% under optimal conditions.
- It enjoys enhanced performance consistency and reduced bank contention during memory-intensive operations, such as multitasking, handling large datasets, and utilizing the integrated GPU.
- It is an effective method to enhance capacity; for instance, to obtain a single 16GB module, dual-rank designs are employed (most 16GB DDR4 modules are dual rank, as current 8Gbit chips require eight to create 8GB of single rank, necessitating 16 chips for 16GB, which equates to two ranks).
- The disadvantage is that it places a greater burden on the memory controller, potentially limiting its ability to achieve aggressive clock speeds compared to single-rank configurations. Additionally, on certain platforms, it may be necessary to lower the maximum speed of dual rank modules when all memory slots are occupied.
Ultimately, the distinction between single and dual rank should be determined by your overall memory configuration and usage scenario. Dual rank modules are increasingly prevalent; for instance, many 16GB DDR4 DIMM modules are dual rank due to the challenges of achieving 16GB in a single rank with current memory technology, although exceptions are emerging with newer, higher-density chips. Manufacturers such as Micron (Crucial) have produced 16GB single-rank DDR4 modules utilizing higher-capacity Rev. B memory chips, which are featured in kits like the Ballistix Max. Generally, 8GB modules are typically single rank, while 16GB modules are usually dual rank, and 32GB modules can often be either dual rank or quad rank.
When an additional option is available to the user, such as the presence of both single rank and dual rank versions of the same kit in the market, the following considerations apply: opting for dual rank may yield superior performance, though the difference is relatively minor. If you plan to implement a multi-module configuration or are constructing a system with an emphasis on overclocking, a single rank may offer more consistent results. For the majority of users, since it is often not feasible to directly choose the rank number of the module—given that manufacturers do not always provide clear information and sometimes require examination of the memory chip layout—there is little need for excessive concern regarding this matter; nonetheless, understanding the distinction can be beneficial.
How to Select RAM Capacity and Speed?
The two primary factors to consider when selecting RAM are capacity (measured in GB) and speed (expressed in MHz, including latency values). The approach to determining the appropriate RAM capacity and speed is contingent upon the user's requirements and system specifications. Below, we have outlined the key topics to consider for making an informed decision:
- Purpose of Use: The nature of your computer usage significantly influences the amount of RAM required. For instance, 8-16 GB of RAM is typically adequate for light tasks such as office applications and web browsing, while 16 GB serves as a suitable baseline for gamers, and 32 GB is recommended for advanced gaming and activities like streaming. Professional tasks (e.g., video editing, 3D rendering, big data analysis) may necessitate 32 GB or more of RAM. Servers or systems operating multiple virtual machines may require 64 GB or greater. To assess your needs, it is advisable to consult the recommended memory specifications of the applications you utilize.
- Motherboard and CPU Support: It is essential to consider the maximum memory capacity and frequency supported by your hardware. The number of DIMM slots on your motherboard, the maximum RAM capacity it can accommodate, and the supported frequencies are critical factors. For instance, many contemporary motherboards can support up to 128 GB of RAM, whereas laptops or older desktops may be restricted to 16-32 GB. Additionally, it is advisable to consult the motherboard’s QVL (Qualified Vendor List) for memory frequency; some motherboards can operate with RAM modules exceeding 3600 MHz without issues, while others may experience instability above 2933 MHz. On the processor front, the CPU also has support limitations, particularly since the memory controller is integrated into the processor. For example, Intel's 10th generation Core processors officially support up to DDR4-2933, while AMD Ryzen processors can officially accommodate DDR4-3200 (though higher speeds are generally accessible through XMP). ECC support is another important consideration: if you intend to utilize ECC memory, ensure that your CPU and motherboard combination are compatible.
- Balance (Capacity vs Speed): When purchasing RAM, it is advisable to determine the capacity requirement first, followed by an examination of speed and latency values. In most instances, having adequate capacity in a system is more critical than achieving high speeds. For instance, when running multiple applications simultaneously or engaging in a large photo or video editing project, opting for 16 GB of 2400 MHz RAM rather than 8 GB of 3600 MHz RAM will yield a significantly smoother experience. Insufficient capacity forces the system to utilize disk swap space, a solution that is considerably slower than even the fastest RAM. Conversely, once a certain capacity threshold is reached (such as 16 or 32 GB), acquiring RAM with a higher MHz rating can enhance performance in specific scenarios. In summary, prioritize meeting your capacity needs before seeking the highest speed and lowest latency combination within your budget. It is important to note that the benefits of very high-frequency RAM may manifest as diminishing returns; for example, the transition from 3200 MHz to 3600 MHz can yield noticeable improvements, while the shift from 4000 MHz to 4400 MHz may result in minimal differences in practical use. The optimal approach is to strike a balance between capacity and speed.
- Multi-Channel Utilization: Contemporary systems incorporate the concept of memory channels (refer to dual-channel, quad-channel). In essence, memory bandwidth can be enhanced by employing multiple memory modules. In a dual-channel configuration, two modules operate concurrently, effectively doubling the theoretical memory bandwidth. Quad-channel, which utilizes four modules (typically found in high-end desktop and server platforms), provides even greater bandwidth. Consequently, rather than relying on a single high-capacity module, utilizing two or four modules, when feasible, to augment capacity positively influences performance. For instance, installing 2x8 GB instead of 1x16 GB enables higher memory speeds due to dual-channel operation. Provided that the total capacity and speed remain consistent, it can be asserted that dual-channel configurations are advantageous, particularly in systems with integrated GPUs. Ensure that your modules are installed in equal pairs according to the channel architecture supported by your motherboard.
- Timing Values (Latency): When assessing memory speed, both the MHz value and timing metrics, such as CAS latency (CL), are crucial. Consider two distinct memory modules: one is DDR4-3200 CL16, and the other is DDR4-3200 CL18. While both operate at 3200 MHz, the CL16 module exhibits lower latency in each operation compared to the CL18 module, rendering it faster. In summary, a lower CL value is preferable; however, comparisons across different frequencies can be complex, as actual latency is typically calculated using the CL/frequency formula in nanoseconds. When selecting memory, opting for a kit with the lowest possible timings can provide a marginal advantage, particularly for processor-intensive games and certain sensitive applications. Nonetheless, the impact of capacity and frequency on overall system performance often outweighs the significance of the CL value.
- Compatibility and Brand Preference: Certain high-frequency memory kits may be optimized for specific platforms, such as those designated as “AMD Optimized.” Consulting the QVL list provided by your motherboard manufacturer for tested memory models can mitigate potential issues. Selecting reputable and reliable manufacturers will also prove advantageous over time. Brands like Corsair, G.Skill, Kingston (HyperX/FURY), Crucial, and TeamGroup are well-established in the memory sector. Their modules typically utilize chips from leading manufacturers such as Samsung, SK Hynix, or Micron, which are prominent DRAM chip producers. Consequently, it is prudent to consider both the technical specifications and feedback from the hardware community when selecting memory.
Taking the aforementioned factors into account, you can ascertain the capacity and speed that best align with your usage requirements. For instance, if you engage in both gaming and content creation, a configuration such as 32 GB DDR5-6000 CL36 represents a well-rounded option, providing both substantial capacity and impressive speed for contemporary demands. Conversely, for an individual whose activities are limited to office tasks and basic multitasking, even 16 GB DDR4-3200 CL22 may suffice. By judiciously selecting RAM that balances your budget and requirements, you will optimize the efficiency of your system.
Conclusion: Factors to Consider When Selecting Memory
In this article, we presented comprehensive insights into various topics, including the distinctions between ECC and non-ECC memory, the concept of memory rank (single rank versus dual rank), and the selection of RAM capacity and speed. Additionally, we examined the products and technologies offered by prominent memory manufacturers in the industry, such as Samsung, HPE, Micron (Crucial), and SK Hynix. Given this information, what factors should we consider when selecting memory, and how can we identify the most appropriate RAM for our systems? Here are some concluding summaries and recommendations:
- Conduct a Needs Analysis: Begin by defining the purpose for which you require a system. Whether for gaming, professional tasks, server management, or everyday use, each scenario necessitates distinct memory requirements. Tailor the capacity and speed recommendations provided in this article to suit your specific needs.
- Maintain Sufficient Capacity: It is essential to avoid bottlenecking your system due to insufficient RAM. Therefore, prioritize generous capacity. For instance, if you are uncertain, opt for 16GB rather than 8GB; choosing 32GB over 16GB will mitigate the risk of future regret. RAM capacity is crucial for effective multitasking and handling large applications.
- Consider ECC Requirement: For home users or gamers, ECC memory is not a necessity, as most likely, your system does not support it. However, if you are developing a mission-critical application, server, or data science workstation, ECC memory should be considered. In any scenario where fault tolerance is crucial, ECC can significantly enhance reliability.
- Rank and Module Configuration: Employing dual modules rather than single modules, or quad modules when feasible, enhances bandwidth (dual-channel/quad-channel). If the capacity remains constant, it is important to note that dual rank modules may offer a slight performance advantage, although the number of ranks is not paramount. Architectures such as AMD Ryzen, in particular, may gain from a dual rank and dual channel configuration. However, avoid excessive population of the system to maintain stability; consult your motherboard's specifications.
- Speed and Timing Selection: It is generally advisable to select the highest speed memory that aligns with your budget and motherboard compatibility; however, it is important to recognize that the advantages of exceedingly high frequencies are limited. A mid-range speed (3200- 3600MHz for DDR4, 6000-6400 MT/s for DDR5) coupled with reasonably low latencies (CL16-18 for DDR4, CL30-36 for DDR5, for instance) will suffice for most users. Unless there is a specific requirement, it may be more prudent to enhance capacity rather than invest significantly in kits that exceed these specifications.
- Brand and Chip Preference: Manufacturers like Samsung, SK Hynix, and Micron provide high-quality DRAM chips. Opting for reputable brands in memory modules also mitigates risks. However, it is important to note that memory modules typically adhere to comparable manufacturing standards, so any module that fulfills your requirements will suffice. Nonetheless, it is prudent to consult product reviews to identify any persistent issues.
- Consider future possibilities: If your motherboard has vacant DIMM slots, contemplate the potential for future RAM upgrades. It may be challenging to locate the same module later, so aim to purchase sufficient capacity initially or distribute modules across four slots rather than opting for dual-channel configurations (for instance, 4x4 instead of 2x8, although this remains dual-channel). If you are investing in new technologies (e.g., transitioning to DDR5), be aware that initial iterations may experience teething issues, and superior kits will emerge over time; exercise patience, but do not hesitate if necessary.
In conclusion, memory selection presents both technical and practical challenges. With the insights provided in this guide, you are now equipped to understand ECC RAM, the distinctions between single and dual rank, and the significance of capacity and speed. This knowledge empowers you to make informed decisions regarding your system and to approach upgrades or new RAM purchases with greater awareness. It is essential to recognize that RAM is as critical a component as the processor and storage; any imbalance or inadequacy can adversely impact your overall experience. By selecting appropriately, you will enhance the performance and stability of your computer.
For an enjoyable and seamless computing experience, ensure that you select a memory capacity and speed that aligns with your requirements. If you find yourself uncertain or seeking guidance for a particular application, user reviews from comparable systems on hardware forums can provide valuable insights. Although technology evolves continuously, the fundamental principles endure: an appropriate quantity of dependable and high-speed memory will always constitute a vital component of systems.